Liquid fluoride thorium reactor
April 18, 2014 |
Liquid fluoride thorium reactor
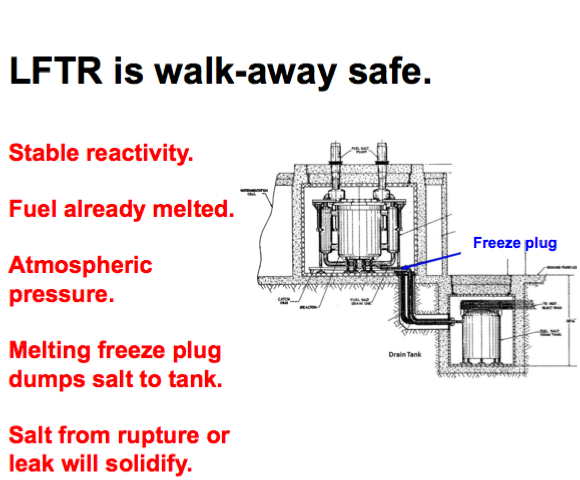
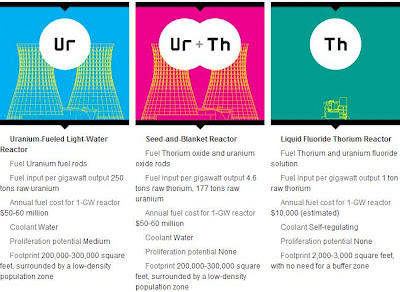
LFTR is a type of thorium molten saltreactor. Molten-salt-fueled reactors (MSRs) supply the nuclear fuel in the form of a molten salt mixture. They should not be confused with molten salt-cooled high temperature reactors (fluoride high-temperature reactors, FHRs) that use a solid fuel. Molten salt reactors, as a class, include both burners and breeders in fast or thermal spectra, using fluoride or chloride salt-based fuels and a range of fissile or fertileconsumables. LFTRs are defined by the use of fluoride fuel salts and the breeding of thorium into uranium-233 in the thermal spectrum.
In a LFTR, thorium and uranium-233 are dissolved in carrier salts, forming a liquid fuel. In a typical operation, the liquid is pumped between a critical core and an external heat exchanger where the heat is transferred to a nonradioactive secondary salt. The secondary salt then transfers its heat to a steam turbine or closed-cycle gas turbine. This technology was 1st investigated at the Oak Ridge National Laboratory Molten-Salt Reactor Experiment in the 1960s. It has recently been the subject of a renewed interest worldwide. Japan, China, the UK and private US, Czech and Australian companies have expressed intent to develop and commercialize the technology. LFTRs differ from other power reactors in almost every aspect: they use thorium rather than uranium, operate at low pressure, fuel by pumping without shutdown, use a salt coolant and produce higher operatingtemperatures. These distinctive characteristics give rise to many potential advantages, as well as design challenges.
Th-232, U-235 and U-238 are primordial nuclides, having existed in their current form for over 4.5 billion years, predating the formation of the Earth; they were forged in the cores of dying stars through the r-process and scattered across the galaxy by supernovas. Their radioactive decay produces about half of the earth’s internal heat.
For technical and historical reasons, the three are each associated with different reactor types. U-235 is the world’s primary nuclear fuel and is usually used in light water reactors. U-238/Pu-239 has found the most use in liquid sodium fast breeder reactors and CANDU Reactors. Th-232/U-233 is best suited to molten salt reactors.
In a nuclear power reactor, there are two types of fuel. The 1st is fissile material, which splits when hit by neutrons, releasing a large amount of energy and also releasing two or three new neutrons. These can split more fissile material, resulting in a continued chain reaction. Examples of fissile fuels are U-233, U-235 and Pu-239. The 2nd type of fuel is called fertile. Examples of fertile fuel are Th-232 and U-238 (mined uranium). Often the amount of fertile fuel in the reactor is far bigger than the amount of fissile, but it cannot be fissioned directly. It must 1st absorb one of the 2 or 3 neutrons produced in the fission process, which is called neutron capture, then it becomes a fissile isotope by radioactive decay. This process is called breeding.
All reactors breed some fuel this way, but today’s solid fueled thermal reactors don’t breed enough new fuel from the fertile to make up for the amount of fissile they consume. This is because today’s reactors use the mined uranium-plutonium cycle in a moderated neutron spectrum. Such a fuel cycle, using slowed down neutrons, gives back less than 2 new neutrons from fissioning the bred plutonium. Since 1 neutron is required to sustain the fission reaction, this leaves a budget of less than 1 neutron per fission to breed new fuel. In addition, the materials in the core such as metals, moderators and fission products absorb some neutrons, leaving too few neutrons to breed enough fuel to continue operating the reactor. As a consequence they must add new fissile fuel periodically and swap out some of the old fuel to make room for the new fuel.
In a reactor that breeds at least as much new fuel as it consumes, it isn’t necessary to add new fissile fuel. Only new fertile fuel is added, which breeds to fissile inside the reactor. In addition the fission products need to be removed. This type of reactor is called a breeder reactor. If it breeds just as much new fissile from fertile to keep operating indefinitely, it is called a break-even breeder or isobreeder. A LFTR is usually designed as a breeder reactor. Thorium goes in, fission products come out.
Reactors that use the uranium-plutonium fuel cycle require fast reactors to sustain breeding, because only with fast moving neutrons does the fission process provide more than 2 neutrons per fission. With thorium, it is possible to breed using a thermal reactor. This was proven to work in the Shippingport Atomic Power Station, whose final fuel load bred slightly more fissile from thorium than it consumed, despite being a fairly standard light water reactor. Thermal reactors require less of the expensive fissile fuel to start.
There are two ways to configure a breeder reactor to do the required breeding. One can place the fertile and fissile fuel together, so breeding and splitting occurs in the same place. Alternatively, fissile and fertile can be separated. The latter is known as core-and-blanket, because a fissile core produces the heat and neutrons while a separate blanket does all the breeding.
Oak Ridge investigated both ways to make a breeder for their Molten Salt Breeder Reactor. Because the fuel is liquid, they are called the “single fluid” and “two fluid” thorium thermal breeder molten salt reactors.
The one-fluid design includes a large reactor vessel filled with fluoride salt containing thorium and uranium. Graphite rods immersed in the salt function as a moderator and to guide the flow of salt. In the ORNL MSBR design a reduced amount ofgraphite near the edge of the reactor core would make the outer region under-moderated, and increased the capture of neutrons there by the thorium. With this arrangement, most of the neutrons were generated at some distance from the reactor boundary, and reduced the neutron leakage to an acceptable level. Still, a single fluid design needs a considerable size to permit breeding.
In a breeder configuration, extensive fuel processing was specified to remove fission products from the fuel salt. In a converter configuration fuel processing requirement was simplified to reduce plant cost. The trade-off was the requirement of periodic Uranium refueling.
The MSRE was a core region only prototype reactor. The MSRE provided valuable long-term operating experience. According to estimates of Japanese scientists, a single fluid LFTR program could be achieved through a relatively modest investment of roughly 300–400 million dollars over 5–10 years to fund research to fill minor technical gaps and build a small reactor prototype comparable to the MSRE.
The two-fluid design is mechanically more complicated compared to the “single fluid” reactor design. The “two fluid” reactor has a high-neutron-density core that burns uranium-233 from the thorium fuel cycle. A separate blanket of thorium salt absorbs the neutrons and its thorium is converted to protactinium-233. Protactinium-233 can be left in the blanket region where neutron flux is lower, so that it slowly decays to U-233 fissile fuel, rather than capture neutrons. This bred fissile U-233 can be recovered by simple fluorination, and placed in the core to fission. The core’s salt is also purified, 1st by fluorination to remove uranium, then vacuum distillation to remove and reuse the carrier salts. The still bottoms left after the distillation are the fission products waste of a LFTR.
One design weakness of the two-fluid design was the necessity for a barrier wall between the core and the blanket region, a wall that would have to be replaced periodically because of fast neutron damage. Graphite was the chosen material by ORNL because of its low neutron absorption, compatibility with the molten salts, high temperature resistance, and sufficient strength and integrity to separate the fuel and blanket salts. The effect of neutron radiation on graphite is to slowly shrink and then swell the graphite to cause an increase in porosity and a deterioration in physical properties.(p13) Graphite pipes would change length, and may crack and leak. ORNL chose not to pursue the two-fluid design, and no examples of the two-fluid reactor were ever constructed.
The recovery of high-purity uranium-233 has been raised as a potential nuclear proliferation concern. A design with no Protactinium separation would ensure that any U-233 is contaminated with U-232 whose decay chain emits 2 MeV gamma rays too hazardous for weapons workers.
A two fluid reactor that has thorium in the fuel salt is sometimes called a “one and a half fluid” reactor, or 1.5 fluid reactor. This is a hybrid, with some of the advantages and disadvantages of both 1 fluid and 2 fluid reactors. Like the 1 fluid reactor, it has thorium in the fuel salt, which complicates the fuel processing. And yet, like the 2 fluid reactor, it can use a highly effective separate blanket to absorb neutrons that leak from the core. The added disadvantage of keeping the fluids separate using a barrier remains, but with thorium present in the fuel salt there are fewer neutrons that must pass through this barrier into the blanket fluid. This results in less damage to the barrier. Any leak in the barrier would also be of lower consequence, as the processing system must already deal with thorium in the core.
The main design question when deciding between a 1/1.5 fluid or two fluid LFTR is whether a more complicated reprocessing or a more demanding structural barrier will be easier to solve.
The LFTR with a high operating temperature of 700 degrees Celsius can operate at a thermal efficiency to electrical of 45%. This is higher than today’s light water reactors that are at 32–36% thermal to electrical efficiency.
The Rankine cycle is the most basic thermodynamic power cycle. The simplest cycle consists of a steam generator, a turbine, a condenser, and a pump. The working fluid is usually water. A Rankine power conversion system coupled to a LFTR could take advantage of increased steam temperature to improve its thermal efficiency. The subcritical Rankine steam cycle is currently used in commercial power plants, with the newest plants utilizing the higher temperature, higher pressure, supercritical Rankine steam cycles. The work of ORNL from the 1960s and 1970s on the MSBR assumed the use of a standard supercritical steam turbine with an efficiency of 44%, and had done considerable design work on developing molten fluoride salt – steam generators.
The LFTR needs a mechanism to remove the fission products from the fuel salt and recover at least the fissile material. Some fission products in the salt absorb neutrons and reduce the production of new fissile fuel. Especially the concentrations of some of the rare earth elements need to be kept low, as they have a large cross section for neutron capture. Some other elements with a small cross section like Cs or Zr can be tolerated in much higher concentrations, so they may accumulate over years of operation.
Removal of fission products is similar to reprocessing of solid fuel elements, without the need to remove and rebuild the fuel cladding. As the fuel of a LFTR is a molten salt mixture, it is attractive to use pyroprocessing, high temperature methods working directly from the hot molten salt. Pyroprocessing does not use radiation sensitive solvents and isn’t easily disturbed by decay heat. It can be used on the highly radioactive fuel directly from the reactor. Having the chemical separation on site, close to the reactor avoids transport and keeps the total inventory of the fuel cycle low. Ideally everything except new fuel and waste (fission products) stays inside the plant.
http://lifesun.info/liquid-fluoride-thorium-reactor/
On site processing is planned to work continuously, cleaning a small fraction of the salt every day and sending it back to the reactor. There is no need to make the fuel salt very clean, the purpose is to keep the concentration of fission products and other impurities low enough.